APPLN devices
In conventional periodically poled lithium niobate (PPLN) devices, the grating k-vector is constant through the length of the structure. In this case, wide bandwidths can only be achieved by use of short crystals, extremely high intensities (limited by the damage threshold), or use of particular wavelength configurations such as operation at pump-degeneracy.
We overcome these limitations by employing aperiodically poled lithium niobate (APPLN) structures, in which there is a smooth variation of the grating k-vector. By sweeping the period of the grating through the phase matching for different spectral components (i.e. a chirped QPM grating), phase matching over an essentially arbitrary optical bandwidth can be achieved.
This behavior is illustrated in Fig. 1, in a high-gain OPA configuration, for three different spectral components.
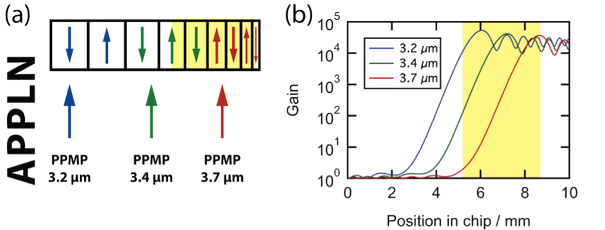
Chirped QPM gratings have been studied since the early days of QPM technology:
- They were initially investigated for broadening of second-harmonic generation (SHG) tuning curves [1-3], and later for frequency conversion involving ultrashort pulses.
- By choice of a suitable QPM chirp profile, the spectral phase of the output pulses can be manipulated, e.g. to achieve pulse compression [4].
- This technique was applied in our group for generation of compressed sub-6-fs blue light [5].
More recently, it has been shown that chirped QPM gratings can also support high-gain, broad-bandwidth OPCPA [6-9]. For example, a linear chirp in the grating k-vector enables a broad, flat parametric gain spectrum. In such devices, each spectral component of the broadband optical pulse is phase matched around a chosen position in the device and experiences exponential parametric gain in the vicinity if this position (see Fig. 1).
Another, recently identified feature of chirped QPM interactions is adiabatic frequency conversion (AFC), which supports a conversion efficiency that increases monotonically in input intensity – in contrast to periodic QPM or birefringently phasematched interactions, which exhibit strong back-conversion after a certain distance.
- AFC was identified in the context of sum frequency generation (SFG) [10].
- Soon afterwards, more general three-wave mixing cases, including OPA, were considered [11]. A detailed theoretical description is given in [12].
This regime offers the potential for ultrabroadand, high-gain, high-efficiency OPCPA in challenging wavelength regions such as the mid-IR.
References
[1] T. Suhara and H. Nishihara,
“Theoretical analysis of waveguide second-harmonic generation phase matched with uniform and chirped gratings,”
IEEE J. Quantum. Electron. 26, 1265 (1990).
[2] M. L. Bortz, M. Fujimura, and M. M. Fejer,
“Increased acceptance bandwidth for quasi-phasematched second harmonic generation in LiNbO3 waveguides,”
Electron. Lett. 30, 34 (1994).
[3] K. Mizuuchi, K. Yamamoto, M. Kato, and H. Sato,
“Broadening of the phase-matching bandwidth in quasi-phase-matched second-harmonic generation,”
IEEE J. Quantum Electron. 30, 1596 (1994).
[4] M. A. Arbore, A. Galvanauskas, D. Harter, M. H. Chou, and M. M. Fejer,
“Engineerable compression of ultrashort pulses by use of second-harmonic generation in chirped-period-poled lithium niobate,”
Opt. Lett. 22, 1341-1343 (1997).
[5] Ref. [145] L. Gallmann, G. Steinmeyer, U. Keller, G. Imeshev, M. M. Fejer, and J. Meyn,
Download“Generation of sub-6-fs blue pulses by frequency doubling with quasi-phase-matching gratings,” (PDF, 82 KB)vertical_align_bottom
Opt. Lett. 26, 614-616 (2001).
[6] K. L. Baker,
“Single-pass gain in a chirped quasi-phase-matched optical parametric oscillator,”
Appl. Phys. Lett. 82, 3841-3843 (2003).
[7] M. Charbonneau-Lefort, B. Afeyan, and M. M. Fejer,
“Optical parametric amplifiers using chirped quasi-phase-matching gratings I: practical design formulas,”
J. Opt. Soc. Am. B 25, 463-480 (2008).
[8] M. Charbonneau-Lefort, B. Afeyan, and M. M. Fejer,
“Competing collinear and noncollinear interactions in chirped quasi-phase-matched optical parametric amplifiers,”
J. Opt. Soc. Am. B 25, 1402-1413 (2008).
[9] Ref. [301] C. Heese, C. R. Phillips, L. Gallmann, M. M. Fejer, and U. Keller,
Download“Ultrabroadband, highly flexible amplifier for ultrashort midinfrared laser pulses based on aperiodically poled Mg:LiNbO3,” (PDF, 387 KB)vertical_align_bottom
Opt. Lett. 35, 2340-2342 (2010).
[10] H. Suchowski, V. Prabhudesai, D. Oron, A. Arie, and Y. Silberberg,
“Robust adiabatic sum frequency conversion,”
Opt. Express 17, 12731-12740 (2009).
[11] C. R. Phillips and M. M. Fejer,
“Efficiency and phase of optical parametric amplification in chirped quasi-phase-matched gratings,”
Opt. Lett. 35, 3093-3095 (2010).
[12] C. R. Phillips, C. Langrock, D. Chang, Y. W. Lin, L. Gallmann, and M. M. Fejer,
“Apodization of chirped quasi-phasematching devices,”
J. Opt. Soc. Am. B, to be published (2013)